Scientific Objectives
Overarching hypothesis: Diabatic processes over North America and the North Atlantic have a major influence on jet stream structure, the downstream development of Rossby waves on the tropopause, and high impact weather phenomena over Europe.
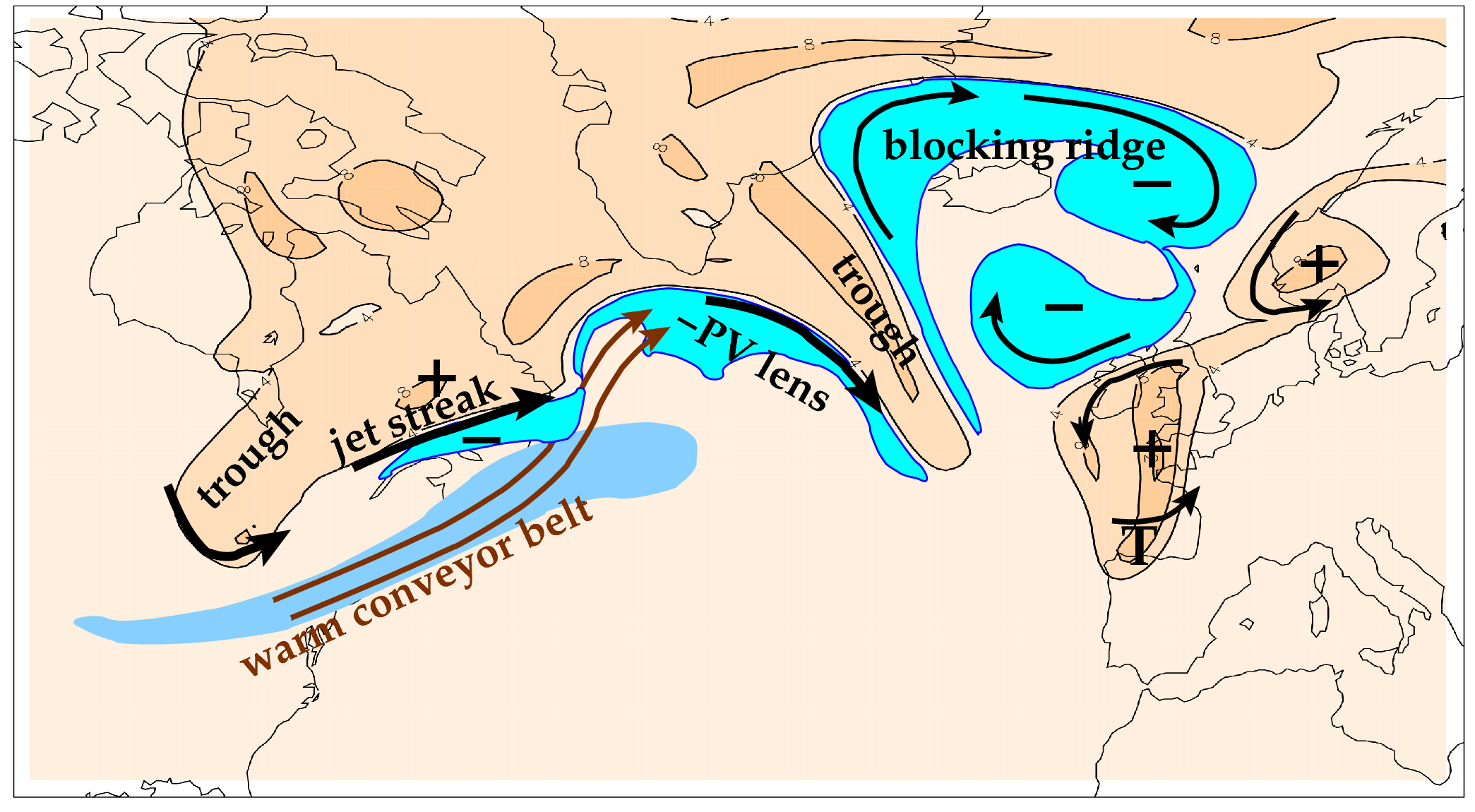
Fig. 1. Illustration of disturbances to the jet stream near the tropopause (orange is stratospheric air; cyan marks upper tropospheric potential vorticity (PV) anomalies). The + and - indicate the sign of PV anomalies. Black arrows show upper-tropospheric wind, boldest where the jet stream is strongest. Brown arrows indicate the motion in a warm conveyor belt which ascends polewards from a tongue of low-level warm, moist air (darker blue). T marks the tip of a cut-off vortex that caused extreme flooding across England as it rotated anticlockwise over the following day (20 July 2007).
A. Diabatic processes and impact on potential vorticity and large-scale dynamics
1. Diabatic processes affecting Rossby wave packets (upscale effect)
a) Warm conveyor belt / latent heating / ridge building
The air in the warm sector of each cyclone flows polewards and ascends in a warm conveyor belt (WCB, Fig. 1) from the boundary layer into the ridge at tropopause level. For North Atlantic cyclones, climatologically the most frequent inflow region of WCBs is along the U.S. East Coast whereas the outflow spreads over the entire central North Atlantic (Madonna et al., 2014). Diabatic processes, such as turbulent fluxes, cloud microphysics and convection in the WCB influence the net heating, the level of the outflow layer and the direction taken by outflow air masses (Martinez-Alvarado et al., 2014a), and has important implications for perturbation growth rates and upscale energy transfer (e.g., Doyle et al., 2014). The effect of the heating on the PV is to strengthen the negative PV anomaly (compared with its surroundings in that layer) in the shallow outflow layer which tends to intensify the upper-level downstream ridge. If the outflow layer is higher, the negative PV anomaly is stronger and more mass enters the anti-cyclonic branch of the WCB flowing into the downstream ridge. The latent heating in WCBs is strong both in the early phase of the ascent when condensation dominates and later when mixed-phase clouds are formed and vapour deposition on snow becomes important (Joos and Wernli, 2012).
b) Long wave radiation / trough reinforcement
A sharp peak in longwave radiative cooling immediately below the tropopause and associated with a step change in water vapour creates a reinforcement of the upper troughs on the stratospheric side in Rossby wave packets (Chagnon et al., 2013). Radiation has also been proposed as an essential process for the formation of so-called tropopause polar vortices (high amplitude cyclonic PV anomalies) in the lower stratosphere (Cavallo and Hakim, 2012), which can disturb the Rossby waveguide from the polar side.
c) PV gradient
The combined effects of the low PV in the WCB outflow, the displacement of the tropopause by the divergent outflow, and increased PV contrast due to radiative cooling lead to the creation of a diabatic PV dipole (positive above and negative below the tropopause), a reinforcement of the horizontal PV gradient and amplification of the Rossby wave PV pattern (Chagnon et al., 2013).
2. Diabatic processes directly involved in high-impact weather events (downscale influence)
a) Rossby wave breaking
Rossby wave breaking leads to PV filamentation forming smaller-scale PV anomalies such as PV streamers and (isolated) cut-off PV vortices (see the cut-off T over Western Europe in Fig. 1). The eastern North Atlantic and Europe are regions where the formation of PV streamers and cutoffs is particularly frequent (e.g., Wernli and Sprenger, 2007), and several studies reported the relevance of these flow features for triggering mesoscale high-impact weather, in particular heavy precipitation (e.g., Martius et al., 2006; Chaboureau and Claud, 2006). Such synoptic wave-breaking events are also important for the large-scale flow itself as they reinforce weather regimes such as blocking ridges like the one shown in Fig. 1 (Michel and Rivière, 2011; Spensberger and Spengler, 2014).
b) Advection of moisture
Large-scale advection of lower-tropospheric existing mesoscale humidity anomalies by PV vortices may lead to heavy precipitation events (e.g., Martius et al., 2006). Moisture advection is also important, e.g., in the inflow region of WCBs. Studies by Schäfler et al. (2011) and Schäfler and Harnisch (2015) have shown that low-level humidity in WCB inflows can be poorly constrained by standard observations, and that errors in the low-level moisture can influence the outflow level of WCBs and thereby the waveguide disturbance and downstream flow evolution. Filaments of moisture within low-level jets in WCBs are important regions of initial condition sensitivity and impact the downstream predictability (Doyle et al., 2014).
c) Frontogenesis, frontolysis, conditional symmetric instability
Extratropical cyclogenesis is accompanied by generation of mesoscale structure by frontogenesis, frontal instability and banding through mechanisms such as conditional symmetric instability initiated in saturated air. For example, such processes are invoked to explain the occurrence of sting jets, damaging winds occurring in the frontal fracture region of Shapiro-Keyser type cyclones (Clark et al., 2005; Gray et al., 2011). However, there have only ever been two research aircraft flights into sting jet cyclones, the latest in 2011 (Martinez-Alvarado et al., 2014b; Vaughan et al., 2015), and the mechanisms shaping the structure of the strongest surface winds are not yet known conclusively. However, the frontal structure associated with sting jets often appears for cyclones that have crossed the large-scale jet from its warm-air to cold-air side (Rivière et al., 2015).
B. Potentially misrepresented diabatic processes in models and impact on the circulation
1. Rossby wave packets
a) Intensity of the negative PV anomaly and PV gradient
The amplitude of ridges is often underestimated in current NWP models (Davies and Didone, 2013). Gray et al (2014) have shown that ridge amplitude decays systematically with lead time in the operational forecasts from the Met Office, ECMWF and NCEP. In addition, the PV gradients decrease with lead time suggesting that models cannot maintain the sharpness of the observed waveguide. One possible explanation is that the PV gradient at the tropopause is smoothed by numerical dissipation in NWP models (via the advection scheme and numerical diffusion) and this has the systematic effect of acting to slow down Rossby waves and decrease their amplitude (Harvey et al., 2015). Another origin of the too weak PV gradient in models might come from the representation of long wave radiative cooling, which is sensitive to the vertical profile of water vapour and cirrus clouds near the tropopause. This has ramifications for forecasts of downstream development, since theory tells us that although both the jet stream maximum and westward propagation rate decrease if the PV gradient lessens, the jet effect is stronger and net Rossby wave phase speed decreases. Perhaps more importantly, Rossby wave packets disperse too rapidly downstream. Therefore, diabatic processes, often operating on small scales, can have important consequences for large-scale forecast error. However, the relative importance of the mechanisms outlined above is not known and the aim of the experiment is to quantify the processes using a combination of new observations and model simulations.
b) Vertical structure of the negative PV anomaly
The negative PV anomaly in warm conveyor belt outflow resembles a thin lens dominated by a static stability anomaly (see e.g., Fig. 1 in the mid-Atlantic). It is hypothesised that forecast models misrepresent the vertical structure of the PV lens which has consequences for the flow "induced" and associated Rossby wave propagation along the waveguide.
c) Displacement of the PV gradient
Ascent, amplified by heating, is associated with horizontal divergence at the outflow level. On each isentropic surface, the divergent flow has a strong component normal to the tropopause PV gradient, resulting in poleward advection of the tropopause. This acts as a "Rossby wave source" and can initiate Rossby wave packets that propagate downstream.
2. High-impact weather, and downscale influence of Rossby wave packets
a) Synoptic-scale upper-level forcing of precipitation events
The advection of mesoscale humidity structures by upper-level PV anomalies gives rise to strong sensitivity of forecasts to the moisture field (Doyle et al., 2014). A better knowledge of the low-level moisture field is required for forecast improvements.
b) Jet streaks
Localised horizontal PV dipoles (such as shown over Newfoundland in Fig. 1) constitute "jet streaks" where the jet stream is more intense. Jet streaks are often initiated as long-lived polar mesoscale vortices (maintained by radiative cooling) approach the jet stream and can have a direct impact on severe weather through strong baroclinic interaction with the lower troposphere.
c) Lower-tropospheric strong winds
It is not clear how lower-tropospheric strong winds are dynamically linked to PV anomalies and what the dominant dynamical balances are for these regions of strong winds. The most damaging winds, like sting jets, have been suggested to emanate from the edge or tip of the hooked cloud shield head on the south-western flank of the cyclone, where evaporation of precipitation occurs. However, the role of evaporation of precipitating bands in the formation of sting jets and in the downward transfer of momentum to the surface is not clear yet (Baker et al., 2014; Smart and Browning, 2014).
d) Connection between lower-tropospheric winds and boundary-layer processes
Destabilization of the boundary layer is required to facilitate downward mixing by convective plumes (Parton et al., 2009). But it is rather difficult to reproduce such a downward transfer of momentum in NWP models under both a real or idealized framework (Parton et al., 2009; Baker et al., 2014). Generally speaking, the interaction between boundary-layer processes and the balanced flow in extratropical cyclones still raises open questions.
|